The Spitzer Space Telescope and a Survey of Cometary Activity
January 15, 2015
Water, carbon dioxide, and carbon monoxide are the most abundant volatile molecules in comet nuclei, and they control comet erosion. Of the three, carbon dioxide is the most challenging to observe due to absorption in the Earth's atmosphere, and therefore it is the least studied. With the Spitzer Space Telescope, we can survey how much carbon dioxide comets produce through their orbits, and assess its role in cometary activity.
Observing cometary gas with Spitzer
The Spitzer Space Telescope is an infrared optimized observatory in orbit around the Sun. Initially, the observatory carried liquid helium to cool the telescope and its instruments. The last of the helium was exhausted in 2009, and the telescope has since been passively cooled. Most of the instruments are too warm to operate, but the shortest wavelength filters of one camera, the Infrared Array Camera (IRAC), can still observe with sensitivities far exceeding what is possible from current ground-based observatories. Fortunately for us, these wavelengths cover an important region for comet science.
The two currently operating IRAC filters are centered at 3.6 and 4.5 μm, well into the infrared regime of light. Observations of comets with the 3.6 μm filter are dominated by light originating from the dust, whereas the 4.5 μm filter covers both dust and emission bands from two gases: carbon dioxide and carbon monoxide. An example of a comet observed with these two filters is shown in an accompanying figure. A prominent dust tail and a diffuse gas coma are easily seen.
IRAC simultaneously captures both gas emission bands in a single filter. Care must be taken when interpreting these images and attributing the gas coma to either species. Molecule-for-molecule, carbon dioxide's 4.26 μm emission band is 10 times stronger than carbon monoxide's 4.67 μm emission band. Comets typically produce the two gases at about the same rates, and we can usually assume a gas coma observed by Spitzer/IRAC is dominated by carbon dioxide. However, this is not strictly true. We have seen some comets with carbon monoxide production rates far exceeding that of carbon dioxide. Comet 29P/Schwassmann-Wachmann 1 is a notable example [1].
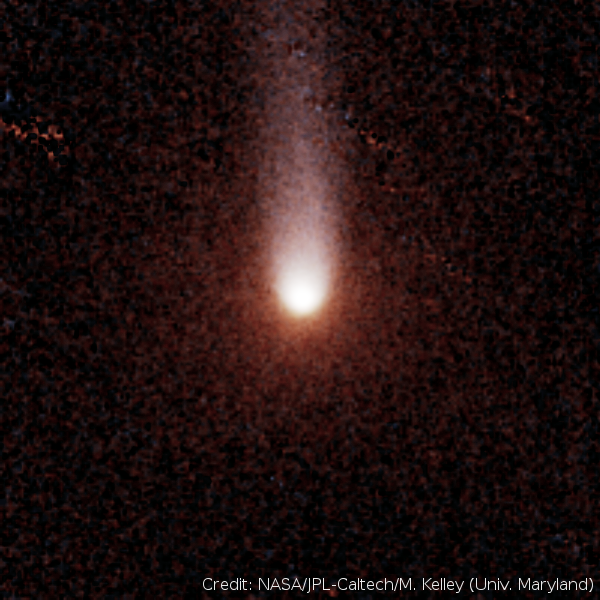
Spitzer/IRAC images of a comet
IRAC images of comet C/2013 A1 (Siding Spring) from March 2014: blue-white corresponds to dust, red-orange to gas. Comet Siding Spring passed Mars at the extremely close distance of 141,000 km in October 2014. Image credit: NASA/JPL-Caltech/M. Kelley (Univ. Maryland)
CO2 orbital trends in comets
A Spitzer Space Telescope survey for cycles 11 and 13, Feb 2015 to Sep 2018.
Abstract: Spacecraft missions to comets return a treasure trove of details of their targets, e.g., the Rosetta mission to comet 67P/Churyumov-Gerasimenko, the Deep Impact experiment at comet 9P/Tempel 1, or even the flyby of C/2013 A1 (Siding Spring) at Mars. Yet, missions are rare, the diversity of comets is large, few comets are easily accessible, and comet flybys essentially return snapshots of their target nuclei. Thus, telescopic observations are necessary to place the mission data within the context of each comet's long-term behavior, and to further connect mission results to the comet population as a whole. We propose a large Cycle 11 project to study the long-term activity of past and potential future mission targets, and select bright Oort cloud comets to infer comet nucleus properties, which would otherwise require flyby missions. In the classical comet model, cometary mass loss is driven by the sublimation of water ice. However, recent discoveries suggest that the more volatile CO and CO2 ices are the likely drivers of some comet active regions. Surprisingly, CO2 drove most of the activity of comet Hartley 2 at only 1 AU from the Sun where vigorous water ice sublimation would be expected to dominate. Currently, little is known about the role of CO2 in comet activity because telluric absorptions prohibit monitoring from the ground. In our Cycle 11 project, we will study the CO2 activity of our targets through IRAC photometry. In conjunction with prior observations of CO2 and CO, as well as future data sets (JWST) and ongoing Earth-based projects led by members of our team, we will investigate both long-term activity trends in our comets, with a particular goal to ascertain the connections between each comet's coma and nucleus.
Team
- Mike Kelley (PI)
University of Maryland, College Park
- Dennis Bodewits
University of Maryland, College Park
- Lori Feaga
University of Maryland, College Park
- Colin Snodgrass
Open University, UK
- Adam McKay
University of Texas, Austin
- Diane Wooden
NASA Ames Research Center
- Matthew Knight
Lowell Observatory
Targets
Comet | Nobs | rmin | rmax | Class | P (yr) | q (AU) | Qmax (x1028/s) | Notes |
---|---|---|---|---|---|---|---|---|
2P/Encke | 14 | -4.1 | 3.9 | ET | 3.3 | 0.3 | 5 | Seasonal jet activity [6]. |
6P/d'Arrest | 3 | 3.1 | 3.9 | JF | 6.7 | 1.4 | 0.4 | |
9P/Tempel 1 | 19 | -4.0 | 3.7 | JF | 5.6 | 1.5 | 2 | Activity peaks pre-perihelion [7]. |
10P/Tempel 2 | 16 | -2.2 | 3.5 | JF | 5.4 | 1.4 | 0.2 | Strong seasonal jet [8]. |
15P/Finlay | 5 | 2.0 | 2.4 | JF | 6.5 | 1.0 | 0.4 | |
19P/Borrelly | 6 | -1.8 | 4.2 | JF | 6.8 | 1.3 | 2 | Strong seasonal jet [9]. |
22P/Kopff | 17 | -2.3 | 4.2 | JF | 6.4 | 1.6 | 4 | Strong seasonal production [10]. |
46P/Wirtanen | 2 | -4.2 | -3.5 | JF | 6.4 | 1.2 | 0.4 | Activity peaks post-perihelion [11]. |
67P/Churyumov-Gerasimenko | 6 | -3.9 | 3.9 | JF | 6.4 | 1.2 | 0.4 | Activity peaks post-perihelion [11]. |
71P/Clark | 12 | -4.6 | 2.6 | JF | 5.5 | 1.6 | 1 | |
73P/Schwassman-Wachmann 3 C | 7 | -4.8 | 2.1 | JF | 5.4 | 0.9 | 2 | Fragmented in 1995. |
73P/Schwassman-Wachmann 3 B | 1 | 2.1 | 2.1 | JF | 5.4 | 0.9 | 2 | Fragmented in 1995. |
81P/Wild 2 | 16 | -3.9 | 3.5 | JF | 6.4 | 1.6 | 1 | Seasonal asymmetry [12]. |
88P/Howell | 7 | 2.6 | 4.4 | JF | 5.5 | 1.4 | 1 | Strong CO2-rich jets. [1, 13]. |
103P/Hartley 2 | 8 | -5.2 | -1.6 | JF | 6.5 | 1.1 | 1 | |
C/2012 K1 (PanSTARRS) | 7 | 2.6 | 8.0 | DN | 1.1 | 7 | ||
C/2013 A1 (Siding Spring) | 8 | 3.0 | 6.9 | DN | 1.4 | 1 | Mars-flyby comet. | |
C/2013 US10 (Catalina) | 13 | -4.0 | 6.5 | DN | 0.8 | 10 | ||
C/2013 X1 (PanSTARRS) | 13 | -4.4 | 6.0 | LP | 1.3 | 10 | ||
C/2014 Q2 (Lovejoy) | 11 | 1.3 | 7.1 | LP | 1.3 | 3 | ||
C/2014 Q3 (Borisov) | 2 | 3.1 | 4.0 | HF | 151 | 1.6 | 0.3 | |
C/2015 V2 (Johnson) | 20 | -5.5 | 3.4 | DN | 1.6 | 15 | ||
C/2015 VL62 (Lemmon-Yeung-PanSTARRS) | 8 | -5.5 | 4.1 | DN | 2.7 | 0.5 | ||
C/2016 M1 (PanSTARRS) | 10 | -6.7 | 2.3 | LP | 2.2 | |||
C/2017 K2 (PanSTARRS) | 3 | -15.5 | -13.7 |
Spitzer observations
0 rows
Comet | Observed (UTC) | Status | rh (au) | tint (s) |
---|
0 rows
Other observations
0 rows
N | Comet | Date (UTC) | rh (au) | Lead | Telescope | Instrument | Status | Filters / Mode | Notes |
---|
0 rows
References
- T. Ootsubo, et al. 2012, AKARI Near-infrared Spectroscopic Survey for CO2 in 18 Comets. The Astrophysical Journal 752, 15.
- D. Osip, D. Schleicher, and R. Millis 1992, Comets - Groundbased observations of spacecraft mission candidates. Icarus 98, 115.
- M. A'Hearn et al. 1995, The ensemble properties of comets: Results from narrowband photometry of 85 comets, 1976-1992. Icarus 118, 223.
- L. Jorda, J. Crovisier, and D. Green 2008, The Correlation Between Visual Magnitudes and Water Production Rates. Asteroids, Comets, Meteors 2008, 8046.
- U. Fink 2009, A taxonomic survey of comet composition 1985-2004 using CCD spectroscopy. Icarus 201, 311.
- Z. Sekanina 1988, Outgassing asymmetry of periodic comet Encke. I - Apparitions 1924-1984. The Astronomical Journal 95, 911.
- D. Schleicher 2007, Deep Impact's target Comet 9P/Tempel 1 at multiple apparitions: Seasonal and secular variations in gas and dust production. Icarus 190, 406.
- M. Knight et al. 2012, A Quarter-century of Observations of Comet 10P/Tempel 2 at Lowell Observatory: Continued Spin-down, Coma Morphology, Production Rates, and Numerical Modeling. The Astronomical Journal 144, 153.
- D. Schleicher, L. Woodney, and R. Millis 2003, Comet 19P/Borrelly at multiple apparitions: seasonal variations in gas production and dust morphology. Icarus 162, 415.
- F. Moreno et al. 2012, Comet 22P/Kopff: Dust Environment and Grain Ejection Anisotropy from Visible and Infrared Observations. The Astrophysical Journal 752, 136.
- D. Schleicher 2006, Compositional and physical results for Rosetta's new target Comet 67P/Churyumov Gerasimenko from narrowband photometry and imaging. Icarus 181, 442.
- D. Schleicher and A. Bair 2011, The Composition of the Interior of Comet 73P/Schwassmann-Wachmann 3: Results from Narrowband Photometry of Multiple Components. The Astronomical Journal 141, 177.
- W. Reach, M. Kelley, and J. Vaubaillon 2013, Survey of cometary CO2, CO, and particulate emissions using the Spitzer Space Telescope. Icarus 226, 777.